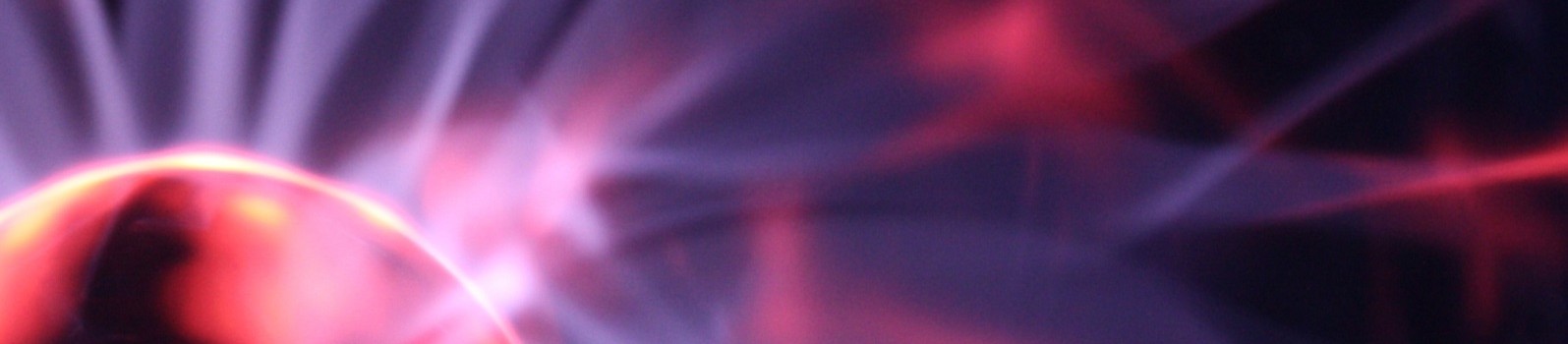
5G and radiation regulations: do we need to raise the bar?
October 30, 2020
Concerns about exposure to electromagnetic fields (EMF) originating from mobile base stations are not new. However, the recent deployment of 5G sites and some of the technological features associated with 5G, such as millimetre waves (mmWaves), have brought the discussions about EMF emissions back to the forefront.
The features that suggest that the impact of EMF resulting from 5G will exceed that of previous mobile technologies include:
- the use of mmWaves and resulting network densification
- the adoption of massive Multi-Input Multi-Output (MIMO) techniques and antenna beamforming
- the rise in the number of connected Internet-of-Things (IoT) devices.
Before discussing the impact of these features on the scale of EMF exposure, it is useful to review some basic facts and draw a baseline for the level of EMF radiated by mobile base stations and handsets.
Current EMF limits
EMF emissions are typically categorised based on their effect on living cells into ionising radiation and non-ionising radiation. Ionising radiation, such as X-rays, are so energetic that they detach electrons from atoms and molecules in living cells. As such, they are potentially harmful in certain doses. Radio frequencies (RF) used for wireless communications (including mmWaves) fall under the non-ionising EMF category. The established effects of RF frequencies are mainly thermal (heat) effects that are only of concern if the radiation power exceeds certain limits. Maximum RF exposure limits are defined by many international, regional and national authorities. The most widely accepted limits are set by the International Commission on Non-Ionizing Radiation Protection (ICNIRP). The ICNIRP exposure limits have a scientific basis and already include an approximate 50-fold safety margin for the general public. For mobile base stations, exposure limits are typically expressed in terms of volts per metre (V/m). The limits vary with frequency. Taking a 5G system operating at 3.5GHz (most auctioned 5G spectrum) as an example, the maximum exposure limit set by ICNIRP for the general public is 61V/m.
To put things into perspective, Exhibit 1 shows the path loss of a mobile base station (macro site) signal using antenna and radio parameters published by some 5G vendors in field trials. The assumptions made in the calculation are very conservative and represent a worst-case situation. The model assumes a relatively high radiating power of 200 watts (compared to 10-100 watts used for 4G) and line-of-sight conditions. It also does not account for power management by the base station and traffic conditions, which further reduce the average radiated power.
Exhibit 1: Electromagnetic field (EMF) level of a macro base station radiating 200 watts at 3.5GHz [Source: Network Strategies]
Even in this conservative scenario (Exhibit 1), it is obvious that the specified ICNIRP exposure limits are only exceeded in close proximity to the base station (up to six metres in this case). This zone is typically referred to as the exclusion zone. Beyond the exclusion zone the signal decays very rapidly and is further weakened by diffraction, reflections and absorption by walls and other materials in the path. As a result, the vast majority of people in a mobile cell area will be exposed to EMF levels that are far below the recommended exposure limit.
However, what often remains unnoticed, is the exposure impact of consumers’ mobile devices. To conserve battery power, mobile devices adapt their transmit power to channel conditions. The weaker the received signal from the base station, the stronger is the mobile device’s transmitting power. In fact, according to many studies, the total EMF exposure is dominated by the person’s own mobile devices rather than by mobile base station radiation. Hence, putting more pressure on base station EMF limits by reducing their power can lead to the opposite of what is desired, at least for active mobile users and people in their vicinity.
Adoption of massive MIMO and antenna beamforming
One point of concern associated with 5G base stations is the maximum reported output power (up to 200 watts), which is two to three times higher than that of legacy technologies. This arises from the need to power many antenna elements. Together with antenna beamforming, this gives the impression that the EMF radiation of 5G antennas will be focused and higher than legacy technologies. However, the actual impact of 5G antenna radiation is far lower due to the random nature of MIMO and antenna beamforming.
MIMO and antenna beamforming take advantage of multiple antenna elements and signal paths to improve transmission and steer antenna beams where they are needed rather than radiating constantly in a wide angular section (Exhibit 2). The radiation pattern is highly dynamic, and an assessment of average EMF levels is only possible using statistical models. This takes into account the spatial distribution of users and traffic characteristics, which change over time and space. Existing evidence based on statistical modeling shows that in about 95% of the time the average radiation power of 5G sites is substantially lower than the theoretical maximum. This is also supported by recent field measurements on a 4G site capable of MIMO and beamforming in Australia. The field tests showed that EMF levels in the vicinity of the base station were 80-90% lower than predicted values using conservative models based on static beam conditions. As such, despite the potential increase in total radiated power, the expected exposure of 5G sites will likely be comparable or lower than that of legacy technologies.
Exhibit 2: Massive MIMO and antenna beamforming employs multiple transmitters and antenna elements to transmit simultaneous data streams, intelligently target users, reduce interference and extend reach. [Source: Network Strategies]
Use of mmWaves and the densification of 5G sites
Another controversial aspect of 5G is network densification, that is, the dense deployment of 5G sites. This comes as a result of using mmWaves, which have a relatively short range, to achieve high data rates. Intuitively, in a very dense network of small 5G cells the RF power is reduced to the level necessary to cover the small service area and limit interference with neighbouring cells. These small cells typically radiate less than 1 watt of power, compared to 200 watts of power radiated by the large coverage macro site analysed in Exhibit 1. The question whether a dense deployment of small low power cell sites will lead to more EMF exposure compared to coverage with a smaller number of high power macro sites, has already been addressed and modelled by some researchers. Evidence shows that the overall EMF exposure is actually minimised when the 5G network is densified.
The other aspect of densification is the use of high frequencies in the mmWave bands. Exposure limits up to 300GHz (including mmWaves) are already covered by guidelines from ICNIRP. It is important to note that mmWaves are subject to significantly higher propagation losses compared to frequencies used by legacy technologies and 5G in the 3.5GHz band. These frequencies have also very low penetration capabilities through walls and human skin. This limits their deployment to serve traffic-demanding hotspots such as airports, stadiums and streets in highly dense urban or business areas. Otherwise, 5G will be largely realised using the sub-6GHz band.
The rise of connected IoT devices
Another controversial aspect is the perception that the massive deployment of IoT devices connected to 5G will increase EMF exposure. Machine-to-Machine (M2M) and IoT devices are not new and are already connected in large numbers to 2G, 3G and 4G networks. Current specifications impose very low limits (lower than 3.2W) for the transmitting power of these devices. However, due to the need to increase battery life, most devices transmit in the range from 25mW to 200mW. Even in the presence of millions of devices in the environment, a distance of a few metres from these devices results in their EMF exposure being negligible. Furthermore, IoT communications in general occur at low data rates and at intermittent or sporadic time intervals.
EMF exposure zone saturation
As with previous technologies, 5G is likely to co-exist with many other legacy technologies with multiple operators sharing the same site. In countries imposing excessive EMF restrictions, far below ICNIRP recommendations, deployment of 5G can be extremely challenging. In these cases, the composite EMF levels from all mobile antennas can easily reach limits that make the exclusion zones unrealistically large for urban deployments. This limits the possibilities of site sharing and can have a severe impact on the quality of service, coverage, cost of deployment and environment. Reducing the output power levels to accommodate the new service is likely to lead to sub-optimal coverage and service quality. Operators usually address this challenge by deploying more mobile base station sites that radiate at a lower power. This not only complicates and increases the cost of deployment, but also has an adverse effect on the carbon footprint of the network. For example, a study conducted on behalf of the GSMA in 2011 estimated that to conform to the strict EMF levels in Brussels, Belgium, 400 new sites need to be built requiring an additional 4,100MWh of energy a year. This corresponds to the average annual electricity consumption of about 500 medium sized households in New Zealand.
Mitigation strategies
There are many ways to mitigate health risks and lessen public concern without imposing stricter EMF limits. From a regulatory perspective, policies that encourage the rationalisation of legacy technologies will reduce total EMF exposure and provide a larger EMF margin for 5G deployment. Regulators should also consider reviewing the existing EMF exposure compliance test standards and adopt methods that are more tailored to the dynamic characteristics of 5G. And most importantly, regulators need to increase awareness of EMF exposure guidelines, and the impact of mobile base stations and mobile devices. One feasible possibility is to deploy RF probes in key locations to measure EMF radiation and share live and past data with the public online.
Total EMF exposure can also be addressed during network planning by selecting a set of base station sites, radio parameters and configurations that minimises EMF exposure while ensuring service quality and economic feasibility. In some respects, the reduction of EMF emissions is also in agreement with the objectives of operators and equipment vendors. Both seek to design and deploy energy-efficient base stations. This trend is progressing rapidly using energy-aware access techniques and communications protocols that will inherently lead to reduced EMF exposure, as the energy will be radiated only when and where it is needed.
It is important to recall that EMF exposure limits recommended by relevant international authorities are based on a substantial amount of scientific research that undergoes regular review, whereas nationally adapted stricter limits are based on cautionary principles to reassure the public or are based on outdated guidelines. In imposing stricter exposure limits, countries may not only compromise the socio-economic benefits of high quality and affordable connectivity, but may end up increasing environmental and health risks.